Sustainable recycling of end-of-life tyres in civil (geotechnical) engineering applications
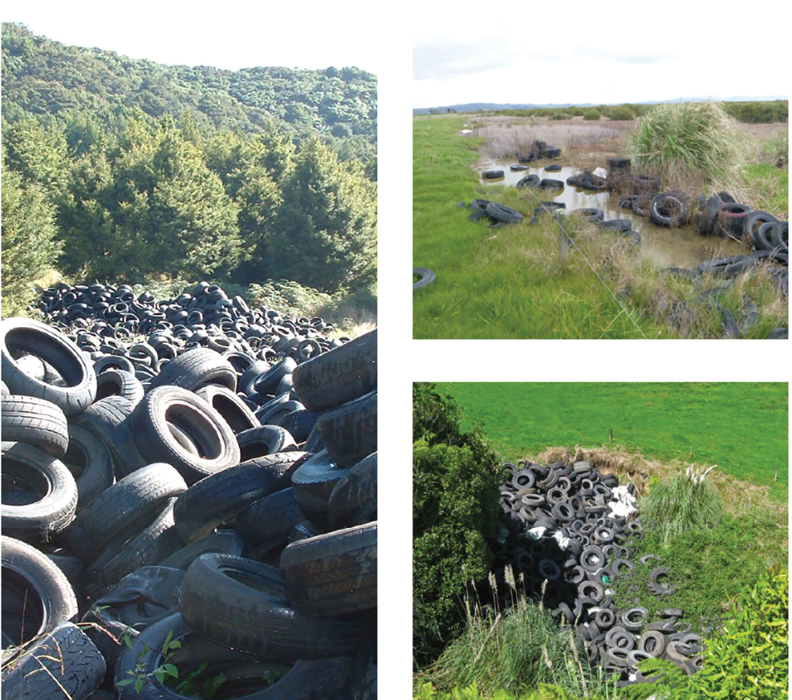
Turning issues into opportunities in the New Zealand context
Abstract
End-of-life tyres (ELTs) are non-reusable tyres in their original form. They enter a waste management system based on product/material recycling and energy recovery or go to disposal. In New Zealand, only 30% of the 5 million ELTs produced yearly are recycled. The residual 70% of ELTs are mainly disposed of through landfill, stockpiles or illegally disposed. However, ELTs cannot be considered as a simple waste to be disposed of. If mechanically sheared into granulated tyre rubber, ELTs may find use in many civil engineering applications. In this paper, key environmental issues associated with ELTs poor management and recycling processes are first described and discussed with reference to the New Zealand context. Then, the potential reuse of ETLs in geotechnical applications is demonstrated based on the results on a multi-disciplinary feasibility study conducted jointly at University of Canterbury and at the Institute of Environmental Science and Research Ltd. (ESR).
Keywords: end-of-life tyres; recycling and reuse of waste materials; gravel-rubber mixtures; sustainable foundation systems
Introduction
Tyre recycling is the process of converting end-of-life or unwanted waste tyres into materials that can be utilized in new products or applications. End-of-life tyres (ELTs) typically become candidates for recycling when they become no longer functional due to wear or damage, and can no longer be re-treaded or re-grooved (Basel Convention Working Group, 1999).
In many countries, ELTs are a controlled waste under environmental regulations, which place a duty of care on waste producers to ensure safe disposal through licensed carriers to licensed sites. For instance, in Europe, since 2003, under the European Union Landfill Directive, waste tyres are prohibited from being used for landfill. Even shredded tyres are not accepted by landfill facilities. In contrast, at present no national regulations are in place in New Zealand to efficiently manage waste tyre recycling, and with the ever-growing volume of ELTs, environmental and socio-economic concerns are urging the reuse of waste tyres through large-scale recycling engineering projects.
Fig. 1. Examples of illegal disposal of ELTs in New Zealand (photos courtesy of Waikato Regional Council)
The issue: improper ELT disposal practices in New Zealand
The current rate of ELT production in New Zealand is over 5 million per year, including passenger vehicle tyres (approximately 4 million) and truck tyres (approximately 1 million), and is expected to grow over time with increased population and number of vehicles on roads. An estimated 30% of such waste tyres are exported or recycled; yet,
the remaining 70% are destined for landfills, stockpiles, illegal disposal or otherwise unaccounted for (Ministry for the Environment, 2015; Cann, 2017), giving rise to piles of waste tyres that do not readily degrade or disintegrate.
Fig. 1 shows typical examples of illegal ELT disposal practices currently seen in New Zealand. The dumping of scrap tyres into open areas and landfills is clearly the least desirable option for the ELT disposal. Vast piles of tyres or buried tyres are unsustainable management practices and cause substantial environmental and health problems. Not only do they occupy a large amount of land (up to 75% void space), but they also harbour many pests, mosquitos and other insects that spread contagious and unknown diseases (Torretta et al., 2015). Potential leaching of metals and other chemicals contained in the tyres is also a danger to the environment as they may be toxic and result in water and soil contamination (Basel Convention Working Group, 1999; Lynch et al., 2017; Banasiak et al., 2019). Another big threat of waste tyres landfilling and stockpiling is the potential for uncontrolled fires. Several tyre fire incidents have been reported in recent years by New Zealand mass media (e.g. Dangerfield 2018a, b and 2019a, b). Though tyres are relatively difficult to ignite, tyre fires are difficult to extinguish and the firefighting and clean-up from tyre fires are costly. More importantly, the gasses emitted from tyre fires are high in CO, CO2, sulphur oxides, and are harmful to both people and the environment (Rowhani and Rainey, 2016). All these serious issues mean that there are great environmental and socio-economic benefits in moving away from waste tyres disposal and to find sustainable management practices.
Waste tyre recycling may be challenging, but it is not impossible to achieve. In Europe, Canada, USA, Japan and many other countries where strategic waste marketing, collection and management procedures have been put in place to effectively making use of recycled ELTs, the disposal of waste tyres has been reduced to 20% or less (Pehlken and Essadiqi, 2005; Torretta et al., 2015; Ministry for the Environment, 2015).
Fig. 2. Waste tyre management hierarchy for the New Zealand context
The challenge: identifying sustainable ways for ELTs recycling in New Zealand
According to the waste management hierarchy (Waste Avoidance and Resource Recovery Act, 2001), reduce, reuse, recycle and energy recovery are the four integrated basic options that should always be considered in dealing with waste problems. To be explicit, the highest priority is to avoid and reduce the generation of waste; when avoiding and reducing waste is not possible, the next most preferred options are to reuse (without further processing) and recycle (processing to make different products) waste materials. This keeps waste in the productive economy and benefits the environment by decreasing the need for new materials and waste absorption. When further recycling is not feasible, it may be possible to recover the energy from wastes, if environmentally acceptable, and feed it back to the economy. Only if waste materials cannot be safely recycled and direct treatment is not feasible, then waste disposal may become a possible management option. In the case of waste tyres, while the avoidance and reduction of the amount of ELTs produced appears to be impracticable – in view of the growing volume of vehicle on the roads – the reuse and recycling of ELTs are certainly viable options, and should be preferred to the energy recovery and disposal (Fig. 2).
In 2015, the Waste Minimisation Fund was targeted at applications that would help solve the problem of ELTs in New Zealand. Although the predisposition in regard to waste tyre management was to reuse and recycle, in its place energy recovery from waste tyres was prioritised. This, however, may be arguably a sustainable solution. That is, tyre derived fuel (TDF) typically has a sulphur content ranging from 0.5% to about 2.0%, and may also contain metals such as lead, cadmium and zinc (Constant and Gosmann, 1997). Therefore, there could be public concerns on the use of TDF and possible toxic air emissions that pose major health risks for the communities living within the vicinity of the industrial units using TDF.
It is clear that only large-scale sustainable initiatives could tackle this nationwide problem, and using ETLs as alternative construction materials in civil engineering applications may be the most promising solution (Fig. 2).
Fig. 3. Example of a multidisciplinary approach used to develop sustainable solutions for ETLs recycling in foundations systems
The opportunity: recycling ELTs in sustainable civil engineering applications
In Australia and New Zealand, geotechnical engineers are increasingly expected to meet requirements for environmentally friendly design, construction and innovation (Arulrajah et al., 2014; Chiaro et al., 2015). In this context, reuse and recycling of construction and demolition materials, commercial wastes and industrial by-products in civil engineering applications are progressively sought as it provides substantial benefits in terms of increased sustainability and reduced environmental impacts.
Specifically to the New Zealand context, taking into consideration the effort is being put into the development of more seismic resilient communities and the expansion of road and railways infrastructures, the reuse of recycled waste tyres in the design of foundation systems that would enhance the seismic performance of residential buildings as well as low-cost light-weight backfill materials for retaining walls and road/railways embankments would be a stimulating opportunity to effectively recycle a large amount of ELTs (Chiaro et al., 2019 a, b).
Such ETLs issues have stimulated research and application of ETLs adoption in an environmentally sustainable way as well as the development of interdisciplinary solutions that require shared knowledge, applied science and advanced experimental and numerical capabilities. Aimed at providing insights that would facilitate the reuse of ELTs in civil engineering applications in the New Zealand context, a joint geo-environmental-structural engineering experimental research program – funded by the Ministry of Business, Innovation and Employment (MBIE) – has been carried out by researchers of the University of Canterbury and the Institute of Environmental Science and Research Ltd. (ESR).
The main components of such research effort consist of:
- Geotechnical and environmental engineering experimental investigations to identify optimum gravel-rubber mixtures (the choice of using of gravel rather than sand is based on performance and implementation considerations), having excellent mechanical properties (e.g. compressibility, stiffness, strength, dynamic properties etc.) and minimal leaching attributes;
- Structural engineering laboratory tests to design fibre-reinforced rubberised-concrete structural elements (e.g. foundation raft, beams and columns) with satisfactory structural performance; and
- Numerical and physical models to prove the concept and evaluate the mechanical performance under static and seismic loads of geotechnical and structural elements individually or as part of a system.
To date, the primary focus of such multi-disciplinary research has been the development of the “eco-rubber geotechnical seismic isolation (ERGSI) foundation systems for medium-rise residential buildings” (Chiaro et al., 2019b; Banasiak et al., 2019; Tasalloti et al., 2020; Hernandez et al., 2020). Nevertheless, the experimental investigations have been designed in a way that they would provide an in-depth understanding of key factors (e.g. rubber content and size, gravel-rubber particles size ratio; rubber-concrete proportion etc.) that affects the mechanical behavior of gravel-rubber mixtures and rubberised concrete, which must be taken into consideration in the design of such materials, therefore, facilitating their adoption also in many other civil engineering applications in addition to ERGSI foundation systems.
In this paper, to benefit the wider New Zealand geotechnical engineering community and stimulate geo-engineers to extend their classical soil mechanics background and think outside the box to come up with safe and sustainable design and construction methods of geo-structures, we synthesise the results of the geotechnical investigations carried out in the Geotechnical Laboratory of the University of Canterbury.
Readers can refer to specific technical publications by the authors, available through the project website (https://sites.google.com/view/ecorubberfoundation/publications), for full details of the “ERGSI foundation systems” project, including findings from environmental and structural investigations as well as results of the numerical investigations as they will become available.
Fig. 4. Examples of reuse of gravel-rubber mixtures in sustainable geotechnical applications
Recycling of ELT-derived rubber in geotechnical applications: sand-rubber vs. gravel-rubber mixtures
In the last two decades, ELT derived materials (in the form of chips, crumbs, granules and shreds) mixed with granular soil (primarily sand) have been used in geotechnical applications, such as light-weight backfill materials for retaining walls and embankments, drainage layers, slope stabilisation and landfill construction (Senetakis et al., 2012; Mashiri et al., 2015). Yet, it was only in recent years, that the attractive strength and dynamic properties of soil-rubber mixtures has regained consideration. Their use has been proposed for isolation backfill material on retaining walls, underground horizontal layer for the mitigation of liquefaction phenomena (Hazarika et al., 2020) and isolation systems with energy dissipation for buildings (Tsang et al., 2019; Chiaro et al., 2019b; Tsiavos et al., 2019). Schematic illustrations are reported in Fig. 4.
To date, much research has focused on the mechanical characterization of sand-rubber mixtures. Nevertheless, in the selection of the soil type and recycled rubber size to form soil-rubber mixtures for use in geotechnical applications, the availability and the cost efficiency of both materials should be carefully considered (Hazarika and Adbullah, 2016). Actually, to avoid inherent segregation of binary mixtures made of large and small particles (Kim and Santamaria, 2008), the recycled rubber should be cut into smaller (sand size-like) pieces when mixed with sandy soils, which will inevitably increase the implementation costs. Hence, it has been recommenced to use gravel-rubber mixtures instead.
Taking into consideration the advantages of using gravel-rubber mixtures over the sand-rubber blends as above described and considering that in New Zealand it is already a common practice to replace the topmost liquefiable sandy soil deposits or problematic soil layers with compacted well-graded gravel layer as part of foundation systems for residential buildings, the adoption of the gravel-rubber assortments in geotechnical applications seems the most appropriate. Yet, such materials have been poorly characterised so far and detailed studies on their physical properties, compaction characteristic and mechanical behaviour were therefore required.
Geotechnical properties of gravel-rubber mixtures
When mixed with gravel, both the rubber content by mass or volume as well as the relative grain size between rubber particles and gravel grains (i.e. aspect ratio or the ratio of median particle sizes; D50R/D50G) plays an important role on the physical properties and mechanical behavior of gravel-rubber mixtures (GRMs).
Hence, in the ERGSI feasibility study, the test materials consisted of a washed pea gravel with rounded grains (G) and two coarse-sized granulated recycled rubber types – i.e. large rubber (RL) and small rubber (RS) – that are free of steel wire and fiber reinforcements. The particle size distribution (PSD) of G, RL and RS with their photos are presented in Fig. 5, while their index properties are summarized in Table 1. The specific gravity (Gs) of G, RL and RS was measured as 2.72, 1.15 and 1.14, respectively. The size ratio (i.e. D50,R/D50,G) between RL particles and G grains is 4/6 = 0.67 and between RS and G particles is 2/6 = 0.33.
Several mixtures prepared at various volumetric rubber content (VRC) of 10%, 25%, and 40% (range of mixtures of interest in foundation engineering applications) by mixing RL and RS with G were also tested. Note that, VRC is defined as the ratio of the rubber particle volume (VR) to the total volume of solid particles (rubber and gravel) (VTOT = VR + VG, where VG is volume of gravel grains):
Dry unit weight of GRMs
For satisfactory performance of structures made of gravel-rubber mixtures (GRMs), it is necessary to properly control the compaction and correctly evaluate the physical properties of compacted SRMs. Similar to conventional soils, laboratory compaction tests represent the best method to understand the sensitivity of compaction to the properties of GRMs (shape and distribution of grains, mineralogy, water content etc.) and to the given actions (compaction methodology and energy).
For the case of gravel-rubber mixtures vibratory table tests were found to be ineffective for compacting the tested materials due mainly to energy absorption ability of the rubber. On the other hand, Proctor compaction tests resulted a viable testing procedure, although the moisture content was not a controlling parameter. Obtained results are reported in Fig. 6. Essentially the dry unit weight of the mixtures decreases linearly with increased VRC, from about 17.2 kN/m3 for pure gravel to 13.2 kN/m3 for mixtures with VRC = 40%. It is worth mentioning that, slightly higher values were obtained for the mixtures with smaller aspect ratio due to smaller rubber particles filling the gaps between the large gravel particles. This is consistent with previous studies on sand-rubber mixtures (Kim and Santamarina, 2008).
Fig. 5. Particle size distribution and photos of gravel and rubber tested in this study
Table 1. Index properties of tested gravel and rubber
Based on the above, one of the benefits of mixing rubber and gravel would be that the in-situ overburden stresses associated with the unit weight of the mixtures could be greatly reduced. For example, the reduction would be of 6% for VRC = 10%, 13% for VRC = 25% and 23% for VRC = 40%. This is expected to lead to reduced design performance requirements, in terms of bearing capacity and settlement, for natural soil deposits that underlie a GRM layer installed as part of a residential structure foundation system. Moreover, thinking of other potential geotechnical applications, it would reduce the earth pressure behind retaining walls or induce less settlement underneath gravel-rubber embankments placed on compressible soils.
Fig. 6. Variation of maximum dry unit weigh of GRMs.
Permeability of GRMs
Permeability of GRMs can be related to the porosity of the material that depends on the PSD of the rubber and host gravel as well as the vertical stress applied. Experimental evidences have showed that typically the hydraulic conductivity coefficient of GRMs with large rubber particles varies between that of typical gravelly soils at low confining stress levels and that of sandy soil at higher confining stress levels. For mixtures of small rubber particles and VRC > 10%, the hydraulic conductivity coefficient is similar to that of sandy soils irrespective of the confining stress level applied.
Hazarika et al. (2020) have shown that the use of GRMs (with VRC < 40%) placed on the top of liquefiable sandy soils can substantially reduce the pore water pressure generation in the sandy soil deposit, the reasons being: (1) GRMs themselves are not liquefiable and therefore can be conveniently used to replace the top most portion of liquefiable soil layers, and (2) having an hydraulic conductivity higher than the sand, GRMs provides a preferential pathway for water to easily flow upwards and quickly dissipate pore water pressure.
Compressibility of GRMs
Compressibility or the capability of GRMs to decrease in volume when subjected to an applied load is one of the most important parameters required in design considerations. For conventional soils having rigid particles such as sand and gravel, any change in volume is due the movement, rotation and rearrangement of non-compressible particles (Lee et al., 2007). The compressibility of GRMs, however, is completely different to that of granular soils, due to significant differences in the elastic modulus of the rigid particles of the host gravel and that of the soft rubber particles. Moreover, under normal stress, not much volume change is associated with individual particle of rubber (Poisson’s ratio of pure rubber ≈ ≈ 0.5), and distortion is the main phenomenon that occurs in pure rubber specimens while being loaded.
A summary of two series of one-dimensional compression tests (k0-lateral stress condition or zero lateral strain) with creep conducted using a newly-developed medium-size oedometer cell (height = 150 mm and diameter = 250 mm) is reported in Fig. 7. In such tests a creep of 30 minutes (which was sufficient to achieve negligible settlement under sustained vertical load under the adopted testing conditions) was applied at each incremental load stage of 100 kPa as well as the measured vertical stress was corrected for the loss of transferred load due to soil-wall friction that was experimentally determined.
The curves reported in Fig. 7 refers to specific values of volumetric strain for various mixtures and applied vertical stress levels of relevance for geotechnical applications. It can be observed that, in general, the vertical strain increases with increasing VRC and applied vertical stress. However, the use of smaller rubber particles induces larger vertical strain under the same stress and VRC conditions.
In the design of geotechnical structures made of GRMs, the two charts reported in Fig. 7 can be used in the selection of optimum mixtures that would meet allowable volumetric strain requirements for any given vertical stress level. It is important also to mention
that if preloaded, over-consolidated GRMs will undergo much smaller volumetric strains that those subjected to normal consolidation.
Fig. 7. One-dimensional compesasion characteristic of GRMS
Fig. 8. Friction angle of GRMs
Strength properties of GRMs
The shear strength of any soil is the result of friction and interlocking between particles, and possibly cementation or bonding at particle contacts. Due to interlocking, particulate materials may expand or contract in volume as they are subject to shear strains. Alike conventional soils, shear strength is one of the most important characteristics contributing to the performance also for GRMs.
Results of direct shear tests conducted on a medium-size apparatus (width = 100 mm; length = 100 mm and height = 45 mm) under three levels of vertical shear stress from 30 to 100 kPa are reported in Fig. 8. In general the friction angle decreases with increasing VRC and vertical stress level. However, it is evident that for the range of VRC tested, the friction angle of GRMs is always greater than 35o, irrespective of the level of vertical stress applied as well as the VRC and the rubber size used. This suggests that GRMs could be safely used in the majority of geotechnical applications
Stiffness, shear strain degradation and damping properties
The design of typical geotechnical structures such as foundations, retaining walls and slopes subjected to cyclic shear loading conditions (e.g. earthquake or traffic loads) would require the evaluation of the dynamic and cyclic response of GRMs, such as the small-strain shear stiffness (Gmax), shear modulus (G) degradation and damping ratio (D).
Fig. 9. Variation of GRMs small-strain shear modulus with varying VRC and confining pressure
Fig. 10. Shear modulus degradation and damping ratio characteristics of GRMs
Results of bender element tests shown in Fig. 9 indicates that the small-strain shear stiffness of GRMs decreases with increasing VRC and increases with confining pressure. This is consistent with previous studies on sand-rubber mixtures. However, for the same level of confining pressure, the stiffness of GRMs is higher than those of sand-rubber mixtures having the same VRC (Lee et al., 2007).
Additionally, the results of small-strain cyclic triaxial tests show that the shear modulus degradation of GRMs decreases with the addition of rubber into GRMs (Fig. 10). On the other hand, also the damping ratio increases with increasing VRC. The use of small rubber size does not affect the overall response. This is an important finding demonstrating that GRMs will perform better that conventional granular soils under cyclic loading retaining their stiffness and dynamic properties over a larger range of shear strains compared to conventional granular soils. Obviously, this is due to the capability of rubber particle to dissipate energy.
Such findings have been confirmed by small-scale impact load tests conducted on GRMs. In such tests a foundation prototype was placed on a 20 cm thick layer of pure gravel and mixtures with VRC = 10, 25 and 40%. It was found that as more rubber was added into the mixtures, the period of the foundation system shifted toward larger value (base-isolation effect due to the reduction of Gmax) while the amplitude of the peak acceleration decreased (i.e. damping effect due to the increase of D). A conceptual diagram showing such effects is reported in Fig. 11.
Fig. 11. Schematic illustration of base isolation and energy dissipation effects of GRMs in ERGSI foundation systems
Table 2. Mass of granulated rubber and number of ETLs to be reused in geotechnical applications
DISCUSSION
How much recycled rubber (or ELTs) could be reused in geotechnical applications?
As summarised in Table 2, based on the results of this study, it has been estimated that up to 280 kg of rubber (free of steel wire and fibre reinforcements) could be used per cubic metre of GRMs. This would correspond to 43 ETLs. This does not include additional rubber that could be used in the rubberised concrete structural elements.
Concluding Remarks
In New Zealand, approximately 3.5 million of ELTs produced yearly are legitimately or illegally disposed of through landfills and stockpiles. Nevertheless, ELTs cannot be considered as a simple waste to be disposed of. Large-scale sustainable recycling initiatives are crucial to tackle this nationwide problem. A promising solution is to reuse waste tyres as a construction material in civil engineering applications. This paper presented the results of geotechnical investigations of a multi-disciplinary project by which ELTs are reused to design earthquake resistant structures by developing innovative dissipative foundation systems. The results of such investigation show that GRMs with VRC ≤ 40% have satisfactory strength properties, low compressibility and enhanced dynamic properties that make it possible to use GRMs in many geotechnical applications. The lightweight properties will reduce the earth pressure behind retaining walls or enhance the bearing capacity of natural soil deposits beneath foundations. Moreover, GRMs could also be effective as liquefaction mitigation technique due to their gravelly-like hydraulic conductivity properties.
Note that the results reported in this paper are based on the use of uniformly-graded gravel and rubber with maximum particles diameter of approximately 10 mm that could be tested in the laboratory using conventional devices. Yet, as in the Geotechnical Laboratory of the University of Canterbury, we are improving our capability of conducting tests on geomaterial with larger particles, additional tests will be carried out on mixtures of gravel AP40 and rubber with 20 mm particles to verify some of the findings reported in this paper and if required refine the design criteria for GRMs.
Acknowledgements
This research was funded by the Ministry of Business, Innovation and Employment of New Zealand (MBIE Smart Ideas Endeavour Research Grant No. 56289). The authors are grateful to Waikato Regional Council for providing the photos shown in Fig.1. The laboratory assistance of Angela Robinson, Hasara Ranchagoda, Maysen Bloemen and Ryan Thom (FYP UG students) is greatly appreciated. The views and opinions expressed in this article are those of the authors and do not necessarily reflect those of MBIE.
References
Arulrajah A, Narsilio G, Kodikara J and Orense RP (2015) Key issues in environmental geotechnics: Australia-New Zealand. Environmental Geotechnics 6:326-330.
Banasiak LJ, Chiaro G, Palermo A and Granello G (2019) Recycling of end-of-life tyres in civil engineering applications: environmental implications. WasteMINZ 2019 Conference, Hamilton, New Zealand, pp. 15.
Basel Convention Working Group (1999) Technical Guidelines on the Identification and Management of Used Tyres. Basel Convention on the control of transboundary movements of hazardous wastes and their disposal, Document No. 10.
Cann G (2017) NZ’s tyre mountains keep growing in absence of recycling scheme. The Press (https://www.stuff.co.nz/environment/92515136/nzs-tyre-mountains-keep-growing-in-the-absence-of-recycling-scheme, accessed 22 March 2020).
Chiaro G, Indraratna B, Tasalloti SMA and Rujikiatkamjorn C (2015) Optimisation of coal wash–slag blend as a structural fill. Ground Improvement 168(1): 33-44.
Chiaro G, Palermo A, Banasiak LJ and Granello G (2019a). Direct shear behaviour of gravel-granulated tyre rubber mixtures. Proc. 13th
ANZ Geomechanics Conference, 1-3 April, Perth, Australia, 221-226.
Chiaro G, Palermo A, Granello G, Tasalloti A, Stratford C and Banasiak LJ (2019) Eco-rubber seismic-isolation foundation systems: a cost-effective way to build resilience. Proc. 2019 Pacific Conference on Earthquake Engineering, 4-6 April, Auckland, New Zealand, pp. 8.
Constant D and Gosmann D (1997) Tire derived fuel use in cement kilns. GCI Tech Notes 3(9).
Dangerfield E (2018a) Cause of massive North Canterbury tyre fire ‘unknown’, investigation to begin. The Press (https://www.stuff.co.nz/the-press/news/101790124/hundreds-of-tyres-on-fire-in-north-canterbury, accessed 22 March 2020).
Dangerfield E (2018b) Community asked to call for Government to combat tyre stockpiling through legislation. The Press (https://www.stuff.co.nz/the-press/news/north-canterbury/101908978/community-to-call-for-central-government-to-combat-tyre-stockpiling-through-legislation, accessed 22 March 2020).
Dangerfield E (2019a) Tyre pile in Amberley, North Canterbury, still a risk a year after huge fire. The Press (www.stuff.co.nz/national/110835486/tyre-pile-still-a-risk-a-year-after-huge-fire, accessed 22 March 2020).
Dangerfield E (2019b) Number of stockpiled tyres underestimated by Environment Canterbury. The Press (https://www.stuff.co.nz/the-press/news/north-canterbury/111551658/number-of-stockpiled-tyres-underestimated-by-environment-canterbury, accessed 28 March 2020).
Hazarika H and Abdullah A (2016) Improvement effects of two and three dimensional geosynthetics used in liquefaction countermeasures. Japan Geotech Soc Special Publ, 2(68): 2336–2341 (2016).
Hazarika H, Pasha SMK, Ishibashi I, Yoshimoto N, Kinoshita T, Endo S, Karmokar AK, Hitosugi T (2020) Tire-chip reinforced foundation as liquefaction countermeasure for residential buildings. Soils and Foundations DOI: 10.1016/j.sandf.2019.12.013
Hernandez E, Palermo A, Granello G, Chiaro G and Banasiak L (2020) Eco-rubber seismic-isolation foundation system, a sustainable solution for the New Zealand context. Structural Engineering International
3(2): 192-200.
Kim HK and Santamarina JC (2008) Sand-rubber mixtures (large rubber chips). Canadian Geotechnical Journal 45: 1457-1466.
Lee JS, Doods J and Santamarina JC (2007) Behavior of rigid-soft particle mixtures. Journal of Material in Civil Engineering, 19(2):179-184.
Lynch P, Gabolinscy C, Tyler M, Turner N, Caldwell J, Kim N, Latham R, Kirkham A, Middleton N, Weiss S, Zaman N, Carter T, Kinaston P, Alebardi M and Ayling J (2017) Guidance for storage and stockpiling end of life tyres for local go vernment. Report 5629, Waikato Regional Council, pp.14.
Mashiri MS, Vinod JS, Neaz Sheikh M and Tsang HH (2015) Shear strength and dilatancy behaviour of sand–tyre chip mixtures. Soils and Foundations 55(3): 517.528.
Ministry for the Environment (2015) Waste tyres economic research. Report 3, May 2015, pp. 87.
Pehlken A and Essadiqi E (2005) Scrap Tire Recycling in Canada. Technical report, MTL 2005-08(CF).
Rowhani A and Rainey TJ (2016) Scrap tyre management pathways and their use as a fuel – a review. Energies 9, 888; doi:10.3390/en9110888.
Senetakis K, Anastasiadis A and Pitilakis K (2012) Dynamic properties of dry sand/rubber (SRM) and gravel/rubber (GRM) mixtures in a wide range of shearing strain amplitudes. Soil Dynamics and Earthquake Engineering 33(1): 38-53.
Tasalloti A, Chiaro G, Palermo A and Banasiak LJ (2020) Effect of rubber crumbs volumetric content on the shear strength of gravelly soil in direct shear apparatus. Proc. of Geo-Congress 2020, 25-28 Feb., Minneapolis, Minnesota, USA [ASCE Geotechnical Special Publication, 319: 259-266].
Torretta V, Rada EC, Ragazzi M, Trulli E, Istrate IA and Cioca LI (2015). Treatment and disposal of tyres: Two EU approaches. A review.
Waste Management 45:152-160.
Tsang HH and Pitilakis K (2019) Mechanism of geotechnical seismic isolation system: Analytical modeling. Soil Dynamics and Earthquake Engineering, 122: 171-184.
Triavos A, Alexander NA, Diambra A, Ibrahim E, Vardanega PJ, Gonzales-Buelga A and Sextos A (2019) A sand-rubber deformable granular layer as low-cost seismic isolation strategy in developing countries: experimental investigation. Soil Dynamics and Earthquake Engineering 125: 1-12.
Waste Avoidance and Resource Recovery Act 2001 No 58
(https://www.legislation.nsw.gov.au/#/view/act/2001/58,
accessed 22 March 2020).